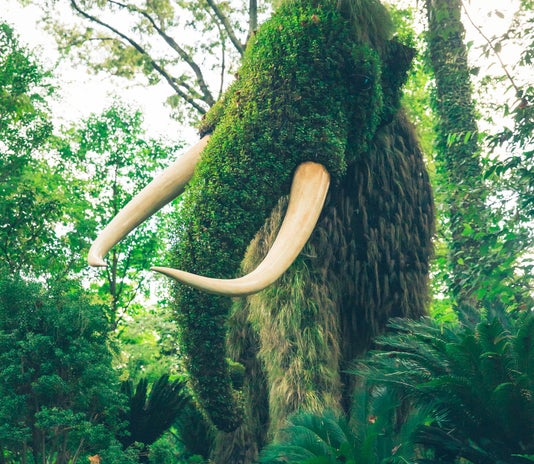
De-extinction is the science of resurrecting extinct species, or their characteristics, through backbreeding, cloning or genetic engineering. This essay, the first in a three-part series, discusses these three techniques, the advantages and disadvantages of each, and how they have been applied to the field of de-extinction.
Backbreeding is a technique that utilises selective breeding methods to concentrate ancient characteristics that have been dispersed over several living breeds into a single lineage. Its simplicity is a great advantage, but it is time consuming and may not be as effective as other methods. Projects using backbreeding include those trying to resurrect the aurochs.
Cloning is a technique that produces genetically identical offspring from a donor. Although cloning may seem advantageous as it retains the genetic identity of the extinct individual, there are many disadvantageous to this method such as limitations on which species are eligible for the technique and the inefficiency of the method. The first de-extinct individual, the bucardo, was produced using this method.
Genetic engineering is a technique that brings together synthetic biology and cloning to produce organisms with altered genomes. In this way, living species can be edited to express the traits of extinct species, resurrecting these traits. This method has advantages over other methods as it opens up de-extinction to older species but in it is still a relatively inefficient method with many uncertainties and difficulties. This is the method being pursued by most de-extinction scientists such as those wishing to resurrect the woolly mammoth.
De-extinction: The Science behind the Fiction
By Ashley Shipley
1 October 2024
1. Introduction:
It should not come as a surprise that we are currently living in a time of environmental crisis on our planet. Global warming leading to melting ice caps and rising sea levels as well as large scale pollution1 have dominated global news stories for years. It is also believed that we are currently living through Earth’s sixth mass extinction. Mass extinction is defined as the loss of 75% of species within a short period of time and is perhaps most associated with the loss of the dinosaurs 65 million years ago2. In the past 500 years, 900 species are known to have become extinct with potentially countless more species whose disappearance has gone unnoticed by us3. 1 000 000 species are currently threatened with extinction, and it is possible that, if things do not change, 50% of all species on planet earth could become extinct in the next 30 years4. It therefore appears that the rate of extinction is increasing5 with estimates of the current rate being at least 1000 times higher than the natural background rate6 and the possibility of 75% of amphibians, bird, and mammals becoming extinct within 500 years2. There are a lot of uncertainties when it comes to future projection and some experts do not believe that we are in the midst of a mass extinction event, however it is undeniable that our current environment is showing uncomfortable similarities to the previous ‘Big Five’ mass extinctions – abnormal climate and atmospheric changes and the presence of ‘high-intensity ecological stressors’2. What is equally undeniable, and what sets our present crisis apart from those that came before, is the cause of these ecological stressors – human beings. Ever since modern Homo sapiens evolved approximately 160 000 years ago7, we have been changing the environment for our own means. From scavenging the Earth’s precious resources to cutting down forests to make space for cities and arable land. We have also exercised dominion over our fellow animals through hunting and domestication. We, as a species, have contributed, in part or in whole, to the decimation of biodiversity on our planet. Should we not be responsible for saving it?
This is the ethos of many of the individuals involved in de-extinction. The discipline of de-extinction was first popularised in Michael Crichton’s 1990 novel ‘Jurassic Park’, but since then there has been considerable debate and lack of consensus over what precisely the goal of de-extinction should be. It is generally understood that the term ‘de-extinction’ is a little misleading. Instead of resurrecting extinct species such as dinosaurs, which Beth Shapiro, a prominent de-extinction practitioner, believes is ‘not possible’5, de-extinction is generally accepted to be the production of an organism that resembles an extinct species in a way that allows for that organism to fill the ecological role once held by the extinct species8. There are individuals whose goal appears to be the cloning of charismatic extinct species such as the woolly mammoth for profit3 but most scientists and companies that are actively involved in de-extinction aim to resurrect specific traits from extinct species which can then be engineered into living species to aid in environmental and species conservation8-12.
This three-part essay series will take an all-encompassing view of the topic of de-extinction. This first essay will review the various techniques that make the science-fiction sounding resurrection of the extinct possible. In the second essay, I will present a balanced view of the highly contentious debate around this discipline, providing you with the ability to evaluate your own opinions on de-extinction. Finally, the third instalment of this series will be an in-depth analysis of several de-extinction candidate species, where I will present criteria for the selection of candidate species and attempt to evaluate how well certain ‘flagship’ species fit within these guidelines.
2. The Science of De-Extinction:
Whatever the motivation behind de-extinction, the scientific techniques involved remain the same and can be grouped into three categories: backbreeding, cloning, and genetic engineering. All three techniques have benefits and limitations and so it is likely that the technique used will be determined by the species to be resurrected.
3. Backbreeding:
Backbreeding, otherwise known as reverse domestication13 or lineage fusion14, is the technique of selectively breeding individuals to produce characteristics present in an extinct ancestral species. Where there is an unbroken lineage from extinct to present day form, the genes that created the characteristics of the extinct species may remain within the living species, although they may be dispersed across various breeds or populations3. The idea behind backbreeding, therefore, is to identify which populations contain the desired traits and selectively breed them together to produce an individual that looks and behaves as the extinct form once did5. These ‘resurrected’ individuals can then be released into the wild to fill the ecological role of their extinct ancestors3.
Traditional backbreeding is similar to selective breeding and domestication in the sense that breeding pairs are chosen based on physical appearance and behaviour and the desired product is a specific look or behaviour reminiscent of the extinct species. Genome-guided backbreeding is a potential alternative to this traditional route in which the genomes of the extinct and living species are sequenced and genes that code for specific traits are identified to make genetically informed choices for mating pairs, increasing the likelihood of success8, 15.
3.1. Advantages:
3.1.1. Simplicity
Although many disciplines such as fossil and isotope studies, archaeology, historical art and genetics can come together to inform practitioners on the characteristics of the extinct population and how best to reinstate this in the present day16, the most basic forms of backbreeding only require reproductive knowledge and an idea of what characteristics are desired.
3.1.2. No direct genetic manipulation
Genetic information could be helpful in the guiding of backbreeding experiments but, unlike other de-extinction techniques, no genetic manipulation is necessary for backbreeding to succeed in the resurrection of extinct traits8.
3.2. Disadvantages:
3.2.1. Lack of genetic identity
A problem with traditional backbreeding is the fact that experiments are based on physical appearance, or phenotype, alone. This means that, even if the experiments do produce an individual who appears and behaves exactly as the extinct species did, the underlying genetics may be different as multiple genes can cause the same phenotypic characteristics10. This difference in genetics between the extinct and de-extinct forms may cause uncertainty over performance8 and the presence of undesirable traits. Without genetic identity, the project may be considered a failure by some, but others see genetic identity as unnecessary, as long as the de-extinct form is able to perform the same ecological role as the extinct form5.
3.2.2. Time consuming
Depending on the species being bred, the speed of backbreeding experiments will vary widely. Many generations of experiments will be required to produce the desired product, this number increasing with the time since extinction and the number of traits to be bred back into the species15. It is possible to decrease the number of generations needed by using the genome-led approach but, although simpler and easier, backbreeding may take longer and require more breeding generations to produce the same results as other techniques5.
3.2.3. Limited number of candidate species
The logic behind backbreeding is that ancestral phenotypes and genotypes can be reconstituted in their modern descendants. For this technique to work, therefore, the extinct species must have living descendants, relatives, or hybrids from which the appropriate characteristics can be ‘bred back’8. This is the case for most domestic species such as cattle, horses, and sheep, as well as some hybrids, but for most extinct species, no sufficiently close relatives exist. A suitable relative would either be a direct descendant of the extinct species, with the speciation event being domestication or hybridisation, or a living species that diverged from the extinct species recently enough for traits to not have evolved or disappeared after this divergence8. These requirements restrict the number of species that backbreeding can be used to resurrect.
3.2.4. Potential hybridisation
It is possible that, if the de-extinct species is released into the same habitat as the modern species from which it has been made, mating may occur between the two species causing hybrid individuals to be produced8. Possible dilution of the target phenotype due to hybridisation may require ongoing artificial selection10 so the long-term maintenance of backbred species may be intrusive and expensive.
3.3. Examples:
Three active back-breeding projects are currently underway to resurrect the aurochs, quagga zebra, and Floreana giant tortoise.
3.3.1. Aurochs
The various projects to resurrect the wild ancestor of modern domestic cattle, the aurochs, are the oldest and most well-known use of the backbreeding technique. Originally a project undertaken by the Heck brothers in 1920s Germany, several teams are seeking a way to resurrect the aggression, size, and physical characteristics of this ancient animal by crossbreeding different breeds of domestic cattle3. These de-extinction projects are being undertaken to fill the ecological niche left unoccupied by the extinction of the aurochs in 1627 by human hunters15. The aurochs was a large herbivore and as such was an important environmental engineer, grazing to produce highly biodiverse grassland habitats. Since the loss of this species, ecosystems with less biodiversity, such as farmland and closed canopy forests, have taken over. The goal of the various aurochs backbreeding programs is to produce a large herbivore akin to the aurochs that can restore these diverse grassland ecosystems throughout Europe3. These projects have seen partial successes in the form of Heck cattle which appear to be more primitive than modern day cattle but smaller and morphologically different compared to the extinct aurochs, and the sequencing of the aurochs’ genome which could aid the experiments5, but as of yet, no cattle sufficiently aurochs-like have been born and interest in these projects appear to be declining12.
3.3.2. Quagga zebra
Quaggas were a close relative of zebras with unique striping, that went extinct in the 1880s due to humans3. A backbreeding project successfully reintroduced this striping pattern into modern zebras, although the colouration of the stripes was incorrect, and there are plans to release these quagga-like zebras into their former habitat to restore the grassland ecosystem, similar to the aims detailed by the aurochs’ de-extinction projects12.
3.3.3. Floreana giant tortoise
Hybrids between the extinct Floreana giant tortoise and other species of tortoise exist, both in the wild and in captivity. Tortoises are effective ecosystem engineers as they graze, disperse seeds, transport nutrients, and are essential for the stability of island ecosystems. The Floreana giant tortoise grazed on, and hence dispersed the seeds of, a wider range of plants than the other species with which it formed hybrids. Although pure Floreana giant tortoises are no longer living, hybrids containing the saddle-backed phenotype that allowed for this more varied diet can be crossbred to enrich and restore the Floreana genome while eliminating the contributions of other species. These now pure tortoises can then be released back to Floreana island in the Galapagos to carry out the same ecological role as their ancestors12.
4. Cloning:
Cloning is the process of replicating the genetic information of one individual to produce an identical individual and is therefore the most logical route to resurrecting extinct organisms. The techniques used for cloning vary for different types of species and each have their own benefits, limitations, and difficulties.
4.1. Mammalian cloning - Somatic cell nuclear transfer:
Somatic cell nuclear transfer (SCNT) is one of the oldest forms of cloning and was brought to the public’s attention in 1996 when ‘Dolly the Sheep’ was successfully cloned using the technique. This technique can be repurposed for de-extinction using the following steps17:
1. The nucleus containing the DNA is removed from an adult cell taken from an extinct organism,
2. The nucleus is removed from an egg cell taken from a close relative of the extinct organism,
3. The extinct nucleus is inserted into the enucleated egg,
4. Electrical impulses are directed to the egg to induce cell division and embryo formation,
5. Once an embryo has formed, it is implanted into the uterus of a close relative of the extinct organism which will act as a surrogate mother,
6. The individual that is birthed by the surrogate will be a clone of the extinct organism from which its DNA originated.
SCNT works by reprogramming the adult nucleus by means of the proteins present in the enucleated egg. Adult cells are fully differentiated meaning that they are unable to produce more than one or two types of cells and so cannot be used to recreate an entire organism. When the nucleus is added to the egg cell, it is reprogrammed or de-differentiated to a pluripotent stem cell, meaning that it can now produce any cell in the body and can therefore form a fully functioning embryo, foetus, and, eventually, offspring5, 10.
4.1.1. Advantages:
4.1.1.1. Genetic identity
Due to the nature of cloning, all offspring will have an identical nuclear genome to the extinct species10. In the minds of some, therefore, cloning constitutes the most reasonable way to produce a truly de-extinct species. As will be discussed later, however, the nuclear genome is not the only thing that determines an organism’s characteristics so, even though the clone will be genetically identical to an extinct individual, it is not guaranteed that this will translate to total identity in terms of behaviour or appearance.
4.1.1.2. Back-up plan for endangered species
There has been success using SCNT on frozen and non-viable cells to produce clones3, 10 which provides the opportunity to form a ‘back-up plan’ for the conservation of endangered species. If conservation efforts to protect and preserve endangered species fail, it may be possible to resurrect the species once they are extinct, provided that cells have taken and frozen prior to death3. Some people see this as the greatest use of de-extinction technology while others consider it to be ethically problematic as the spotlight may be taken away from the conservation of species when they are still alive. These and other viewpoints will be discussed in detail in the second essay in this series.
4.1.2. Disadvantages:
4.1.2.1. Limited to recently extinct species
As cells with a complete, undamaged nuclear genome are required for cloning to succeed3, limitations are placed on exactly which extinct species can be resurrected using this technique. As DNA decay and damage begins to occur immediately after the death of the individual living cells are the best source of a complete nuclear genome so the most likely candidates for SCNT resurrection will be species that have gone extinct recently enough to have had their cells harvested and frozen prior to death10. This covers only a small proportion of extinct species and doesn’t include particularly charismatic, ‘flagship’ species such as the woolly mammoth, so alternative de-extinction techniques will be needed if the goal is to resurrect more long-extinct species. However, as will be discussed in the third essay in this series, guidelines for the selection of candidate species seem to concur that the more recently the species went extinct, the more desirable it is as a candidate for de-extinction. This limitation put on cloning may therefore not be a major disadvantage as it may force practitioners to consider the issues relating to the de-extinction of long-extinct species.
4.1.2.2. Low efficiency
SCNT cloning is highly inefficient in its original form. Only 5-10% of the embryos that are transferred to surrogates in same-species cloning develop to produce offspring. This is even lower for cross-species or interspecies SCNT (iSCNT), which is required for de-extinction, with a 1-6% success rate15. There are several potential explanations for this such as incompatibilities between the nuclear and mitochondrial genomes, incomplete reprogramming, and the use of an inappropriate surrogate mother. In de-extinction iSCNT, the nuclear genome is provided by the extinct species while the mitochondrial genome is provided by the living species that donated the egg. If the two species are not sufficiently closely related the two genomes may be incompatible which can produce unusual phenotypes5 or prevent the development of the clone altogether. This issue could theoretically be overcome through the addition of mitochondria from the extinct species15, but this doesn’t seem possible at the moment5. Another problem common to cloning is the incomplete reprogramming of the somatic cell nucleus. If the nucleus is not correctly de-differentiated by the egg, abortion and development of birth defects are possible. The efficiency with which the egg reprograms the nucleus can be increased by inserting the nucleus into a frog egg or treating it with extracts from one15. Additionally, proceeding through steps 1-4 and stopping embryo development after a few rounds of cell division, growing identical cells in the laboratory and repeating the SCNT process with these cells could increase the efficiency of reprogramming by giving the egg two chances to do it5. Another way to increase efficiency is to avoid the reprogramming step altogether by using embryonic or induced pluripotent stem cells5. Finally, if the surrogate that is used is not a sufficiently close relative or is too different from the extinct species, incompatibilities in size may occur which can lead to problems in gestation and birth for both the surrogate and the offspring18. A potential solution to this is the use of an artificial womb for development of the offspring outside of the body, however this is not currently technically feasibile5.
4.1.2.3. Environmental influences
As previously mentioned, clones will be genetically identical, at least where their nuclear genome is concerned, to the extinct species that donated the DNA. However, this genome is not the only thing that determines how an individual acts or looks. The epigenome is best understood as a range of molecular tags that are attached to the genome that control the expression of genes within cells and so determine the function and appearance of these cells, therefore genetically identical individuals with different epigenomes may have different phenotypes. The epigenome is affected by the developmental environment so if that environment is different in the surrogate compared to the extinct species, the epigenome of the clone will likely be different to that of the extinct species, with the possibility of a different phenotype as a consequence5. Additionally, the community of microorganism of microbiome that lived within the extinct species will not be reconstructed using cloning which also may have an impact on the offspring19. Inheriting the surrogate mitochondrial DNA may also cause differences in phenotype8. Finally, the post-natal environment is likely to be different for the offspring compared to the extinct species. This means that any behaviours that were learnt from older individuals of the species such as foraging, mating, and defensive behaviours, may not be replicated by the new environment causing the offspring, although genetically identical to the extinct species, to behave in ways more akin to their surrogate family than their extinct relatives8, 18. Ultimately, there are likely to be many different environmental influences to phenotype which makes the end product of the cloning of extinct species uncertain.
4.1.2.4. Expensive:
The highly technical and inefficient nature of SCNT makes it a very expensive technique which may or may not be successful in resurrecting extinct species. Many detractors of de-extinction see projects to resurrect extinct species as taking money away from more conventional conservation programs20. This view is perhaps too simplistic, but it is a rational fear and will be evaluated in the second essay in this series.
4.2. Avian cloning – Primordial germ cell transfer:
Owing to difficulties collecting and manipulating bird eggs at an early enough stage of development, SCNT is not possible in avian species. Therefore, an alternative technique using primordial germ cells (PGCs), the precursors to egg and sperm cells, was developed5, 15:
1. Cells from extinct species are reprogrammed to form stem cells,
2. These stem cells are developed into PGCs,
3. The PGCs are injected into a developing egg during the first 24 hours after it has been laid, before the sex organs have fully developed,
4. The injected PGCs from the extinct species and the PGCs from the surrogate move to the developing sex organs where they mature and form gametes – egg and sperm cells,
5. When the egg hatches, the resultant bird will be of the species that laid it (i.e. not the extinct species) but will contain some gametes that contain the DNA of the extinct species,
6. If this bird mates with another similarly manipulated bird, two extinct species gametes may fuse producing a member of the extinct species.
4.2.1. Advantages:
4.2.1.1. More efficient than traditional SCNT
As the reprogramming of the somatic cells is done prior to transfer to the egg, the process is more efficient than traditional SCNT and is reminiscent of the alternative methods used to increase SCNT efficiency. Because of this, experiments should be quicker, less expensive, and less resource intensive than traditional SCNT5.
4.2.1.2. Only way to clone birds
Components of bird eggs such as the hard shell and large yolk combined with the advanced stage of development of the embryo when it is laid, mean that the nucleus cannot be accessed or manipulated in a way that would affect the entire embryo. This means that traditional cloning methods such as SCNT are not applicable in birds5, 8, 15. This is problematic as two leading de-extinction candidates, the New Zealand moa and the passenger pigeon, are birds. Primordial germ cell transfer provides an effective alternative to SCNT which can be used to resurrect these, and other, species.
4.2.1.3. Increased genetic diversity
A problem with cloning in general is the lack of genetic diversity it provides for the offspring. If one somatic cell is used to produce several clones, this population will have no genetic variation and so will have limited adaptability and reduced survival in the wild. Producing PGCs from extinct somatic cells, however, allows for the production of a more genetically diverse population as PGCs contain only half of the total number of chromosomes present in a somatic cell. These haploid cells need to be fused with another haploid cell during fertilisation to produce an embryo so, although the PGCs themselves could be considered clones as they contain the same, albeit it less, genetic information as the extinct individual, the de-extinct individuals produced through mating will not be a clone and so will be genetically different compared to the extinct species and, most importantly, any other individuals produced in this way5.
4.2.2. Disadvantages:
4.2.2.1. Same as for SCNT
The disadvantages of primordial germ cell transfer are generally the same as for SCNT with environmental influences and low efficiency (this time in terms of the rate at which the gametes produced from the injected PGCs are used in fertilisation being poor5) being the main problems with this method.
4.3. Cloning of other animals:
4.3.1. Fish
Stem cell and embryonic nuclear transfer are possible although whether adult somatic cells can be used in this process is unclear15.
4.3.2. Amphibians
Although no adult frog has been produced using traditional SCNT methods, the same alternative methods highlighted for fish may be useful for amphibians15.
4.4. Examples:
Cloning projects for the Tasmanian tiger (thylacine) and gastric-brooding frog have had limited success21, 22 but one project led to the birth of the first ever de-extinct organism: the bucardo.
4.4.1. Bucardo:
The bucardo or Pyrenean ibex was a subspecies of mountain goat that lived in the Pyrenees for 90 000 years before declining throughout the 19th and 20th centuries due to hunting and finally going extinct in 2000. Skin cells were taken from the last remaining bucardo, an elderly female named Celia, in 1999, and in 2003, these cells were used, via somatic cell nuclear transfer, to produce a kid13. This project exemplified the disadvantages inherent in cloning, especially interspecies cloning. The species was only very recently extinct and had been harvested for cells prior to extinction. From a starting point of 154 embryos, only 1 developed to term, constituting a 0.6% success rate23. The clone itself died several minutes after birth due to lung malformation which is not unusual in goats but may have been due to the cloning process3. A hybrid had to be produced between the Spanish Ibex and domestic goat to act as a suitable surrogate13. The project was expensive and ran out of funding before another de-extinction attempt could be comensed23. The fact that the clone had no apparent chance of survival due to its developmental issues has led some people to dismiss this experiment as ‘not a true de-extinction’5. What is certain, however, is that an animal with the same genetic material as its mother was born, three years after its mother, and all other members of its species, had died. This constitutes a huge achievement that provides great hope that cloning can be used to resurrect species.
5. Genetic engineering:
The final category of de-extinction methods is genetic engineering which builds on the cloning techniques discussed in the previous section to enhance their ability to resurrect extinct species, especially those that went extinct hundreds or thousands of years ago and therefore do not have complete nuclei available for cloning. Genetic engineering can be broken down into two main categories: synthetic genomes and genome editing. The latter category can be further broken down to Synthesis and PCR based methods, based on how the DNA that will be edited into the genome is produced.
5.1. Synthetic genomes:
The most seemingly straightforward way of producing an intact genome of a long-extinct individual is by synthesising the entire genome in the laboratory. Theoretically, all you would need to do is sequence the DNA found in bones, hair, or other fossilised remains and use DNA synthesis techniques in the lab to create a fully functioning, yet synthetic, genome. This genome could then be packaged inside of a nucleus and used as the basis for one of the cloning techniques outlined in the previous section, hence producing a de-extinct individual. Practically, there are many challenges with this method.
Firstly, the DNA present in the remains of extinct species, especially long-extinct species, is exceptionally fragmented and degraded. DNA has a half life of 512 years meaning that half of the total DNA present at the beginning will have disappeared or degraded to unusable levels 512 years later24. This means that even the remains of species that died out a few hundred years ago will not contain complete or near complete genomes. The outlook is especially bleak for the poster child of the de-extinction movement, the woolly mammoth, which went totally extinct 3400 years ago24 and whose DNA now exists in highly damaged fragments of 30-90 base pairs each5. Considering the mammoth genome is estimated to be 4.7 billion base pairs in length25, many millions of these fragments will need to be found, isolated, sequenced, and aligned into a genome. This could be particularly problematic for sections of the genome that are highly repetitive but are nevertheless highly important for the functioning of the cell, such as centromeres15. Furthermore, a reference genome taken from a closely related species must be used to align the DNA fragments into a genome. This means that, if a species does not have a sufficiently close relative or if the relative has not had their own genome sequenced, the genome cannot be aligned in order and so will be incomplete and non-functional15. Any genome that is planned to be used alongside cloning techniques must be complete and it is simply impossible to sequence and align the complete genome of any species, never mind extinct ones5.
Even if sequencing of an entire genome were possible, the physical synthesis of this genome represents another major problem. Although synthetic genomes of small organisms such as E. coli and yeast have been successfully produced, the production of the long genomes of vertebrates is still unfeasible. Firstly, synthesis of long strands of DNA is not currently possible so large genomes must be synthesised in smaller strands and then chemically linked inside or outside a host cell. There are challenges to this, however. If this joining occurs outside a host cell, there is a risk of increased instability with increased length so the synthesis of large genomes would be impractical. Longer stretches of DNA can be assembled within host cells such as E. coli and yeast however, the size of some genomes, especially those like the mammoth genome, may simply be too large for these small cells to handle25. Ultimately, it seems that there is no feasible way at present to synthesise large genomes from scratch.
Say it were to become possible to sequence, align, and synthesise a complete genome. As the candidate species for de-extinction are overwhelmingly eukaryotic vertebrates, the next stage would be to separate this genome into chromosomes and envelope these chromosomes in a nuclear envelope to produce a nucleus that can be used for cloning. For long-extinct species, especially those with no sufficiently close relatives, identifying where chromosomes are within the genome or even how many chromosomes the species has is a challenge that may be insurmountable. For a genome to function correctly, it must be correctly separated into chromosomes so if this is not possible, resurrection using this technique is not possible. Furthermore, although there is some knowledge around how to envelope a single chromosome in a nuclear envelope to produce a functional nucleus, there are many unknowns surrounding whether it will be possible to ensure that the nuclear envelope forms around all the chromosomes 15, 25. The stage of nuclear envelopment, therefore, is another challenge along the synthetic genomes de-extinction pathway.
As should be clear from this explanation, the process of creating a fully functional nucleus that can be used for the cloning of an extinct species from scratch is thwart with complications, many of which are impossible to overcome and may remain so even with the development of more sophisticated technologies. Synthetic genomes, therefore, is not a viable de-extinction technique, but there is an alternative that utilises synthetic biology in a more reasonable and feasible way and represents the most attractive method for de-extinction.
5.2. Genome editing:
Genome editing is the practice of removing a section of DNA and replacing it with a different section, either synthesised or isolated from another cell. This method is therefore simpler and more feasible than the synthesis of an entire genome as only the small section of DNA that is to be inserted needs to be synthesised, if that15, 26. The general procedure for genome editing is as follows 3, 5, 8, 12:
1. Sequence the genomes of the extinct species and a close living relative,
2. Compare the genomes and identify differences,
3. Use genome editing technologies such as CRISPR/Cas9 to remove a section of DNA from the relative and replace it with the equivalent section from the extinct species,
4. Use this edited nucleus as the basis of an appropriate cloning technique.
5.2.1. Advantages:
5.2.1.1. No need to sequence the entire genome
The sequencing of the entire genome of the extinct species was a necessity for the synthetic genome method of de-extinction and the impossibility of this step meant that the entire method was impossible to carry out. With the genome editing method, however, it is unnecessary to sequence the entire genome as the extinct genome is not the one being cloned. It would likely be beneficial to have the full genomes of both the extinct and the living relative to have a comprehensive view of all of the differences between the two species and hence the ability to change all of the DNA sequences that differ between the two, however, as previously described, this is not currently possible, and may never by possible for extinct species, and is not necessary for genome editing to commence.
5.2.1.2. Already enveloped in a nucleus
Another major challenge for the synthetic genome method is the process of enveloping the newly formed genome in a nuclear membrane to prepare it for cloning. As the genome that will be cloned is an edited form of the living species, it will already be packaged inside of the nucleus, so this step is avoided altogether.
5.2.1.3. Small amounts of DNA synthesis required
While synthetic genomes require the synthesis of many small DNA sections which will then be chemically linked, it is not necessary to expend so much labour for genome editing. As previously mentioned, there are two types of genome editing: synthesis based and PCR based. Synthesis based genome editing requires the synthesis of small DNA sections that match certain sections of the extinct genome and will then be used to replace the corresponding parts of the living genome. PCR based genome editing works similarly but instead of synthesising the DNA that will be inserted from scratch, the DNA sections from the extinct genome itself can be amplified using a technique called the Polymerase Chain Reaction or PCR. This technique, which is also used in the sequencing stage, takes a small amount of DNA and amplifies it to produce may identical DNA segments. These segments, as long as they are of acceptable quality, can then be inserted into the living genome. PCR based genome editing is both quicker and less expensive compared to synthesis-based genome editing, however both are more feasible than synthetic genomes due to the requirement for much less DNA synthesis 15, 26.
5.2.1.4. Only a few edits are needed
Depending on the goal of a de-extinction project, the number of genome edits required will vary. For example, if the goal of the project is to produce an individual that was genetically identical to the extinct woolly mammoth, many edits will be need as, although more than 98.5% of mammoth and Asian elephant genomes are identical13, this still means that 1.5 million base pairs are different and so must be edited to fully resurrect the species. However, if the goal of the project is to simply resurrect a single trait, for example hairiness, significantly fewer edits will be needed to achieve this. The majority of de-extinction projects have a goal somewhere in the middle of these two extremes with the aim to resurrect several traits to allow a living species to perform the ecological role of an extinct one, in the former habitat of that extinct species. Although this is likely to be quite a challenge, the fact that only a fraction of the total genome needs to be sequenced, synthesised or amplified, and inserted into the living genome is a great advantage of the genome editing method.
5.2.2. Disadvantages:
5.2.2.1. Low efficiency and high cost
Like all of the de-extinction methods discussed in this essay, genome editing suffers from low efficiency and high cost26. Efficiency issues stem from unintended genome cuts by the editing technologies that may cause breaks and insertions at the wrong part of the genome as well as the fact that the CRISPR/Cas9 system is not universal and so can not be used to edit every part of the genome. Furthermore, the rate at which the new DNA strand is incorporated into the genome is lower than repair of the genome through normal cellular mechanisms, so only 0.5-20% of ‘edited’ cells will contain the extinct species DNA sequence. Repair mechanisms can be inhibited to increase this success rate but there is a chance that these inhibitors may cause issues for embryo development15.
5.2.2.2. Difficulty identifying appropriate edit sites
To avoid editing every site which differs between the two genomes, and hence allow a project to move more quickly with less expense and labour, sites must be identified that not only differ between the genomes but also contribute to a desired characteristic in the final organism. The process of identifying these sites is long and likely the most difficult aspect of a genome editing project. The most basic method is through trial and error: making edits and growing the edited cells to see what the effect of these edits were3, 5. This can be incredibly laborious and resource intensive and, especially if the introduction of multiple traits or complex traits that rely on multiple genes is required, may be fruitless as genes do not tend to act individually and the phenotypic effect of one edit may be changed when another edit is introduced. Another method is to use knowledge gained from other species to guide choices. It may be that genes implicated in hairiness in one species may also be involved in the same trait in another species. This is the most sensible method but will still require a great amount of experimentation before the right genes can be found and edited.
5.2.2.3. Limited to certain species
The limitations posed on which species are eligible for genome editing are considerably less stringent than those for cloning but there are still limitations on time since extinction. When DNA fragments become shorter than about 30 base pairs, aligning the fragment on a genome becomes unfeasible. This means that, given the half-life of DNA as previously discussed, the maximum amount of time that could have passed between death and resurrection is about 1.5 million years27. There are many practical issues regarding DNA preservation, however, which means that most species DNA will become unusable after about 100,000 years. There are several examples of individuals who died longer ago than this who have had their DNA sequenced, the oldest being a 700,000-year-old ancient horse. Cold and dry environments, preferably caves or permafrost, are the ideal places for the preservation of DNA so the oldest species that can be resurrected using this method are likely to be found here5.
A further limitation to eligibility is the presence of a close living relative that can act as a reference genome for the aligning of the extinct genome, a template genome for the insertion of extinct species DNA sequences, and a surrogate host for the cloning of the edited nucleus8.
5.2.2.4. Issues with cloning
As the final step of the genome editing method is to ‘clone’ the edited nucleus using an appropriate cloning technique, the disadvantages of cloning also apply to this method, specifically issues surrounding environmental influences and efficiency.
5.2.2.5. Lack of genetic identity
Unless the goal of the project is to change every single difference between two genomes to recreate an individual that is genetically identical to an extinct form, which is perhaps prohibitively difficult and some would say unnecessary, the product of a genome editing project is likely to be more genetically similar to the living host species than the extinct species8. This will be covered in more detail in the second essay in this series, so it is enough to say here that this aspect of de-extinction is a major debating point which some see as a disadvantage of the technique and others see as either an advantage or a neutral feature.
5.3. Examples:
As it is the most feasible method of achieving a successful de-extinction, several projects are using genetic engineering, specifically genome editing, to resurrect extinct species. In this section I will briefly cover two foremost projects: the woolly mammoth and the passenger pigeon.
5.3.1. Woolly mammoth
The woolly mammoth has been brought up several times throughout this essay, and for good reason. It is the species that is the subject of Beth Shapiro’s book ‘How to Clone a Mammoth’5 which has been an invaluable source for this essay and is seen as a ‘landmark project’28. Several teams across the world are trying to resurrect this megaherbivore, the frontrunner being George Church’s company Colossal. This project, instead of trying to resurrect the mammoth in its entirety, aims to use several of the unique traits present in the mammoth to engineer ‘cold-resistant elephants’. This will not only expand the geographical range of the endangered elephant but also potentially succeed in reconstructing the mammoth steppe, the grassland environment maintained by mammoths that once populated the Arctic. The consequence of this could be the prevention of the melting of the permafrost which, if it were to continue, would lead to millions of tonnes of carbon being released into the atmosphere, accelerating global warming and the extinction crisis that we are currently living through28. As of 2016, Church’s lab reported that 45 genetic changes have been made to produce smaller ears, low-temperature-efficient haemoglobin, fur, and subcutaneous fat using elephant cells3. The team is a long way away from producing a fully functioning elephant-mammoth but there is no reason why they should not be able to succeed, at least as far as the science is concerned.
5.3.2. Passenger pigeon
The passenger pigeon was a unique and ecologically important bird species that went extinct in the early 20th century after aggressive hunting. Revive & Restore, a company dedicated to many types of conservation work, are attempting to resurrect this magnificent species using genome editing combined with the PGC transfer cloning method. This project includes sequencing the genomes of the passenger pigeon and its close relative, the band-tailed pigeon, and identifying regions to edit. Primordial germ cells will then be taken from band-tailed pigeons, cultured and edited to contain the appropriate passenger pigeon genes. These edited PGCs will then be injected into a rock pigeon embryo at the right developmental stage and mature to form passenger pigeon gametes. Two ‘edited’ rock pigeons will be bred to produce a pure passenger pigeon which will then be raised by pigeon colonies before they are reintroduced into the wild29. This procedure and the passenger pigeon species will be discussed in greater detail in the third essay in this series.
6. Conclusion:
In conclusion, de-extinction, or more specifically the resurrection of traits present in extinct species, is a possible strategy that could be used, alongside other conservation programs, to increase biodiversity and prevent the mass extinction which many believe is imminent, if not already here. Three techniques - backbreeding, cloning, and genetic engineering - have been identified as the methods by which de-extinction is possible, with genetic engineering through genome editing commonly seen as the most feasible method, although the most appropriate technique varies with the species to be resurrected. Although still a relatively new discipline, several projects are currently underway with the de-extinction of the woolly mammoth, passenger pigeon, dodo, thylacine, aurochs, quagga, and more being pursued by companies and laboratories around the world. This essay has shown that although difficult and complicated by many limitations, it is scientifically possible to resurrect the genes, appearance, and/or behaviour of extinct species. However, is this enough to resurrect the species itself? Some say not. Beth Shapiro, although one of de-extinctions foremost practitioners and proponents, does not believe it is possible to resurrect a long-extinct species, such as the woolly mammoth and what is more, she doesn’t want to. She, and many others in the field, are not pursuing de-extinction in order to produce a genetic replica of the passenger pigeon or the thylacine. They are developing and refining technologies that will enable endangered species to thrive within the habitats of their extinct relatives to reconstitute ecosystems that have become less productive and biodiverse since the extinction of key species. This will provide additional habitats for the endangered species, hopefully reducing extinction pressures, and will protect the environment and therefore other species, including humans. Although these aims seem admirable and beneficial, de-extinction is a highly contentious topic with many disciplines involved in the debate. The next essay in this series will discuss the various viewpoints both for and against de-extinction.
Add comment
Comments